Plenary & Keynote speakers
Spotlight on Visionary Speakers at Droplets 2025
We are thrilled to present an exceptional lineup of plenary and keynote speakers for the Droplets 2025 conference.
These distinguished experts will set the stage for groundbreaking discussions, bringing together diverse perspectives on the challenges and opportunities in the field. Join us as they explore cutting-edge solutions, share visionary insights, and drive meaningful progress in addressing one of the most critical issues of our time.
Plenary speakers
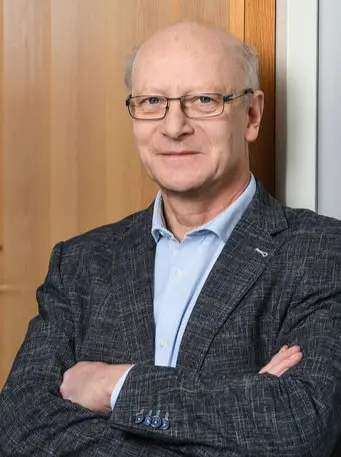
Hans-Jürgen Butt
Max-Planck Institute Mainz, Germany
Hans-Jürgen Butt studied physics in Hamburg and Göttingen. He did his PhD at the Max Planck Institute for Biophysics with Ernst Bamberg, Frankfurt, in 1989.
After a postdoc in Santa Barbara and a researcher position back in Frankfurt, he became associate professor at the University Mainz and three years later full professor in Siegen. In 2002 he joined the Max Planck Institute for Polymer Research in Mainz as a director. His research is on the structure and dynamics of soft matter interfaces in particular wetting and surfaces forces.
Petra S. Dittrich
ETH Zürich, Switzerland
Prof. Petra S. Dittrich is Associate Professor for Bioanalytics at the Department of Biosystems Science and Engineering, ETH Zürich, since 2014. She develops microfluidic devices for bioanalytical and diagnostic applications. Her research interests focus currently on single cell analysis, lipid membrane studies and biomedical applications such as blood-vessels-on-chip. She studied Chemistry at Bielefeld University (Germany) with a semester at the Universidad de Salamanca (Spain). She earned her PhD degree at the Max Planck-Institute for Biophysical Chemistry (MPI Göttingen, Germany) in 2003. After a year as postdoctoral fellow at the MPI Göttingen, she was postdoc at the Institute for Analytical Sciences (ISAS Dortmund, Germany). From 2008, she was Assistant Professor at the Department of Chemistry and Applied Biosciences (ETH Zurich).
For research stays, she visited the Cornell University (Ithaca, USA, in 2002) and the University of Tokyo (Japan, in 2005). She received among others awards the prestigious Starting Grant from the European Research Council (ERC, from 2008-2014) as well as the ERC Consolidator Grant (2016-2021).
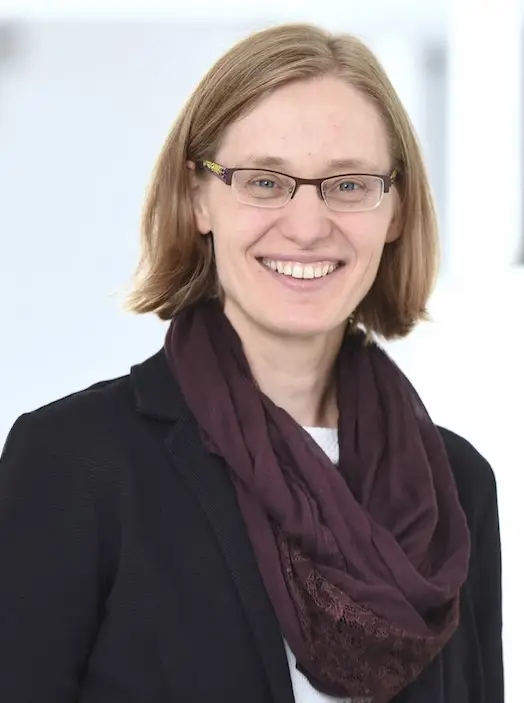
Kripa K. Varanasi
MIT, USA
Kripa K. Varanasi is a Professor of Mechanical Engineering at MIT, Cambridge. He leads an interdisciplinary lab focused on understanding many aspects of physico-chemical transport, and biological phenomena. His work has contributed to the development of innovative materials, devices, products, and processes designed to address challenges in areas such as energy, water, agriculture, transportation, medical devices, and consumer products. He is passionate about entrepreneurship, scale-up, and manufacturing and has translated various technologies from lab to market. He has co-founded several companies, including LiquiGlide, Dropwise, Infinite Cooling, Alsym Energy, AgZen, and Coflo Medical. LiquiGlide has been recognized by Time and Forbes magazines as one of the “Best Inventions of the Year.”
Prof. Varanasi has been honored with several awards, including the NSF Career Award, DARPA Young Faculty Award, SME Outstanding Young Manufacturing Engineer Award, ASME Bergles-Rohsenow Heat Transfer Award, ASME Gustus L. Larson Memorial Award, APS Milton van Dyke Award, and MIT Graduate Student Council’s Frank E. Perkins Award for Excellence in Graduate Advising.
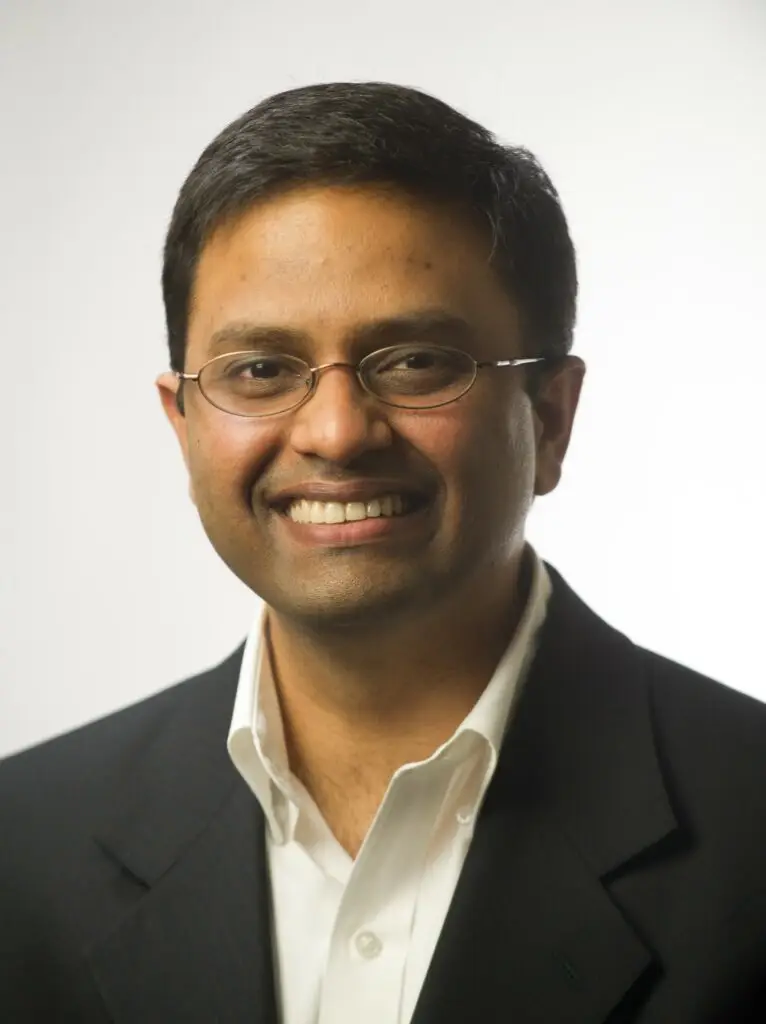
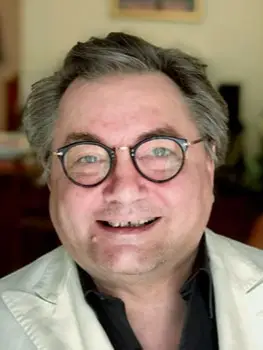
Emmanuel Villermaux
Aix-Marseille Université, France
Manuel Villermaux received his PhD from the University of Paris VI, Pierre & Marie Curie, in Grenoble where he was appointed at CNRS, and where he obtained the habilitation form the Joseph Fourier University. He now holds a position of distinguished Professor at Aix Marseille University, and at the Institut Universitaire de France.
His interests are in the mechanics of deformable bodies in the broad sense, from fluids to solids, with a particular taste for mixing and fragmentation.
He is an associate editor of the Comptes Rendus Mecanique and a founder associate editor of Physical Review Fluids. He is an elected Fellow of APS, of Euromech and has been the recipient of the Bronze Medal from CNRS, of the Edmond Brun Prize from the French Academy of Sciences, and of the Stanley Corrsin Award from APS.
Petia M. Vlahovska
Northwestern University, USA
Petia M. Vlahovska is a Professor of Engineering Sciences and Applied Mathematics at Northwestern University. She received a PhD in Chemical Engineering from Yale University (2003) and MS in Chemistry from Sofia University, Bulgaria (1994). Prior to joining Northwestern University, she was faculty at Dartmouth College and Brown University.
Her research encompasses fluid dynamics, membrane biophysics, and soft matter. Prof. Vlahovska is a Fellow of the American Physical Society (2019). She has been awarded is a Guggenheim Fellowship (2024), National Science Foundation CAREER Award (2009), David Crighton Fellowship from the Department of Applied Mathematics and Theoretical Physics, University of Cambridge, UK (2004), and a Research Fellowship from the Alexander von Humboldt Foundation to visit the Membrane Biophysics Lab at the Max Planck Institute of Colloids and Interfaces (2016).
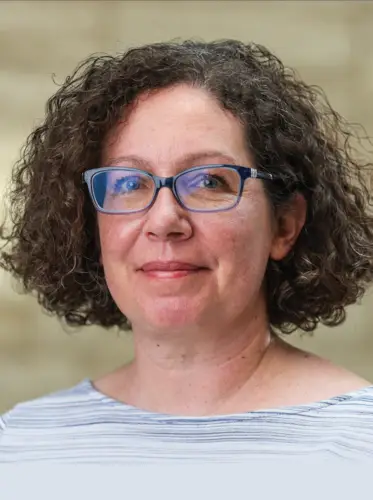
Keynote Speakers
We are excited to announce that the Droplets 2025 conference will feature an inspiring lineup of keynote speakers who will share their expertise and vision for the future:
Catherine Barentin, Université de Lyon (France)
Xu Deng, University of Electronic Science and Technology of China (China)
Burak Eral, TU Delft (Netherlands)
Hanneke Gelderblom, TU Eindhoven (Netherlands)
Jose Manuel Gordillo, Universidad de Sevilla (Spain)
Anne Juel, University of Manchester (United Kingdom)
Djamel Lakehal, Afry (Switzerland)
Dominique Legendre, IMFT (France)
Elise Lorenceau, Université Grenoble Alpes (France)
Jacco Snoeijer, University of Twente (Netherlands)
Tadd Truscott, King Abdullah University of Science and Technology (Saudi Arabia)
Maja Vuckovac, Aalto University (Finland)